Labradorescence
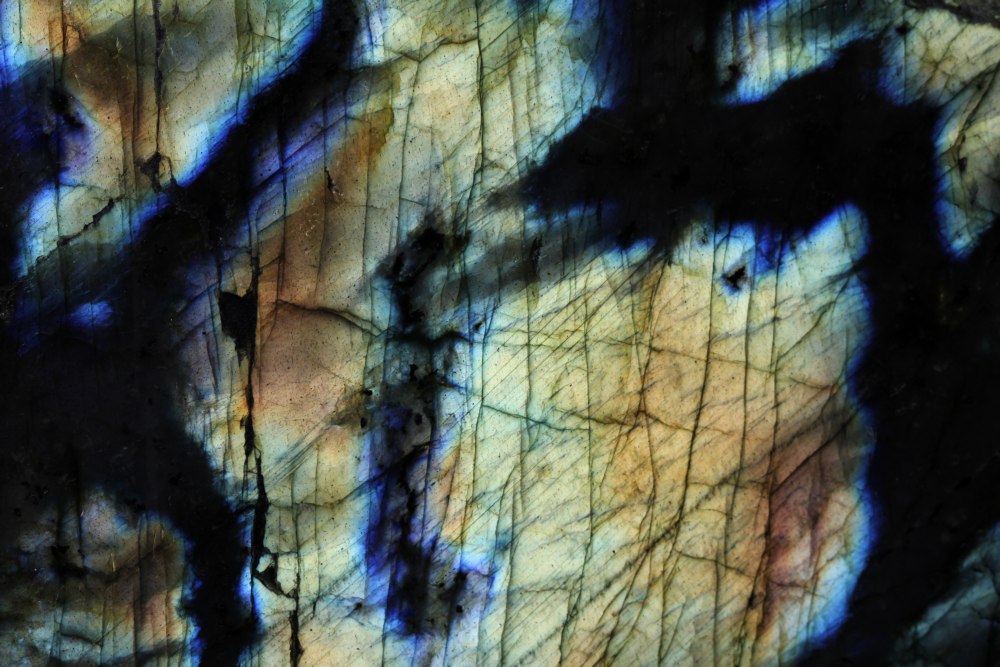
Labradorite is probably my favorite mineral. Ignore for the moment that it’s stunningly beautiful, and check out the killer science:
Labradorescence
The distinctive light refraction known as “labradorescence” takes its name from the feldspar mineral labradorite. Labradorescence is very “deep” and notoriously hard to capture on camera — so go look at some labradorite in real life as soon as you can! (Fun words abound in this category. Similar types of light refraction are called aventurescence and adularescence.)
Particularly sparkly specimens of labradorite are often labeled “spectralite,” but I personally find this annoying since there’s no clear delineation around what is and isn’t spectralite — they’re the same mineral. And anyway spectralite sounds like a kind of rope.
Exsolution
The labradorescence and also, to some degree, the variety of hues found in labradorite, are the result of a fascinating effect called “exsolution,” “phase exsolution,” or simply “phase state instability.” This describes a situation in which the heat and pressure are sufficient to create a crystal, but not quite enough to keep its mineral components dissolved. As a result, the whole rock (the “phase” in geologic terms) holds together, but internally it splits into tiny sheets (called “lamellae”) of different mineral compositions.
Light can penetrate some of these sheets and is refracted in different directions by others, making for a striking display that changes drastically as you move the stone or the light-source. You can read more about exsolution here. Below is a photo showing exsolution in a pyroxene sample, which (unlike labradorite) only exsoludes into two mineral compounds, making the lamellae very easy to see:
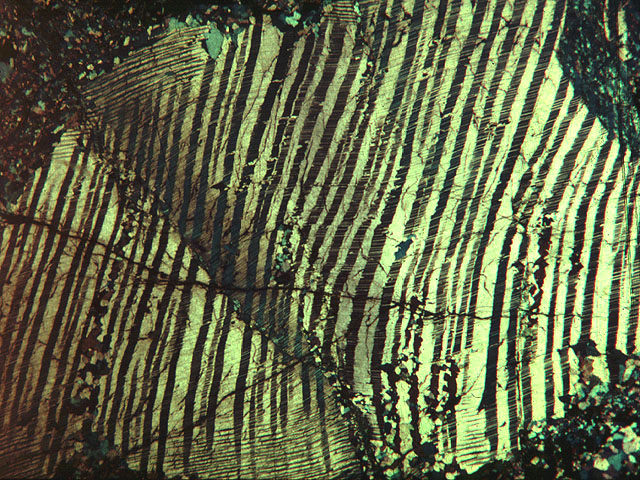
Triclinic Crystal Structure
Now, abandon any thoughts of things being lined up in neat parallel sheets, because labradorite is one of the least parallel types of mineral when it comes to its structure. Labradorite is one of the relatively few minerals that have a triclinic crystalline structure. These are some examples of triclinic crystals:
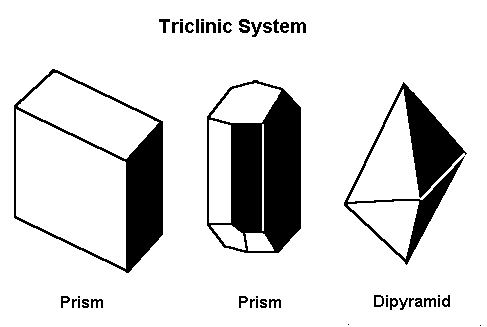
So what’s special about those shapes? Well, this is the class of crystals formed of polygons whose vectors are all of different lengths, and none orthogonal to each other — thus, no ninety-degree angles and no equal-length lines. If you imagine what it’d be like to build with blocks of shapes that met those criteria, you can see that labradorite’s internal structure must be extremely complex.
Thanks to the phase exsolution creating all those fine, light-penetrable layers of different mineral composition, we can see further into this rock than many; and unlike minerals where light passes straight through, labradorite “shows” us where some of the boundaries of its complex internal shapes are. So labradorite looks, when light penetrates it, like it’s filled with random spidery cracks — but they’re not cracks, and they’re not random.
OK, science admired. Let’s look at some more pictures!
[envira-gallery id=”5513″]
Raquel Gaudard
Amazing tie-dye of nature.